Parrots are built for flight.
Before we can explore the physical and psychological effects of clipping wings, we must first discuss some basics of natural history and biology. The purpose of this discussion is to debunk the myths which claim parrots don’t need to fly, don’t want to fly, don’t like to fly, or are bad at flying. These myths have been reinforced within our culture because clipping wings is so common. When people see parrots sitting on perches, never flying, it promotes the incorrect assumption that “parrots don’t really fly”. Even more damaging to a proper understanding of the role flight plays for a parrot is when someone sees a parrot attempt to fly and it falls or crashes instead. The people witnessing these things for the first time often don’t know much about parrots; it doesn’t cross their minds that those birds might be clipped, or that they might have been clipped before learning to fly. Then, if they or someone they know comes to own a bird later on, the idea of clipping wings seems harmless enough (since they think that parrots aren’t very good flyers anyway), and the cycle continues. But this is a cycle we can break if people are given the correct information.
Birds evolved from theropod dinosaurs about 160 million years ago, during the Jurassic period, and are part of the same group which includes well-known dinosaurs like the T-rex and velociraptor, though birds are not direct descendants of either.1 In fact, birds had already taken on some very familiar shapes even before non-avian dinosaurs went extinct 66 million years ago.2 Rest assured, birds have been flying for much, much longer than we have been keeping them as pets.
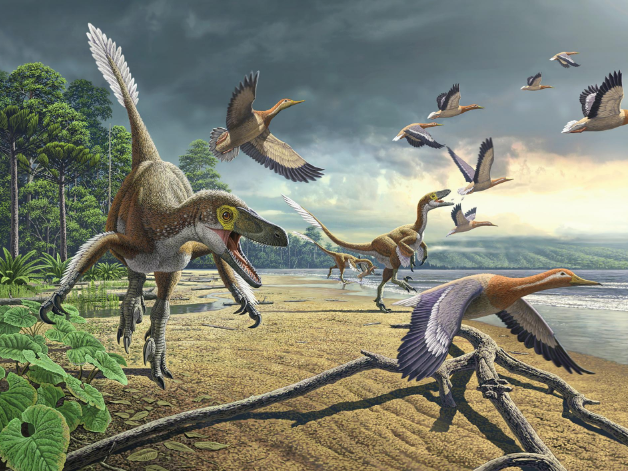
These “ducks” are actually an artist’s depiction of Vegavis iaai, which lived about 68-66 million years ago. Illustration from National Geographic.20
Because flight is such a highly specialised activity, birds have evolved numerous adaptations in order to make it possible. To put it simply, Mother Nature has engineered their bodies for flight.3 A flighted bird’s skeleton, like an aircraft frame, is as light as possible while remaining as strong as possible. It is neither heavy nor fragile. In fact, a flighted bird’s bones are denser than those of a flightless mammal of the same size.4 Strong and stiff compared to their weight, most bird bones are filled with air and reinforcing struts. (Source: see footnote 3.) The length of their wing bones scales more steeply with weight than the length of their leg bones because their wings are meant to support a heavier load.5 Many of their bones are fused together for stability and reduced in some way to decrease weight. (Source: see footnote 3.) Flighted bird species even have a special extension of their breastbone, called the keel, which serves as an anchor point for their extremely developed and powerful flight muscles. (Source: see footnote 3.) The larger pectoralis major pushes the wing down, while the smaller supracoracoideus pulls it up.6 These muscles alone account for 25-35% of total body mass in an average flying bird.7 In humans, all of our muscle combined accounts for 30-40% of our total body mass.8 To say that a volant bird’s flight muscles are important would be an understatement. Flightless birds like the ostrich and the emu, on the other hand, have comparatively larger and more powerful leg muscles.9
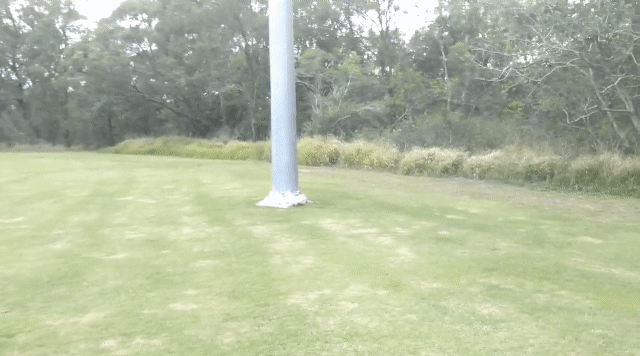
Don’t blink or you’ll miss her! This black-capped lory has been professionally trained to fly outdoors and return (DON’T TRY THIS AT HOME), but she lives in captivity as a companion bird. She gets everything she needs from humans yet flies with this level of enthusiasm everyday. She asks that you please ignore myths you may hear about parrots not enjoying or needing flight. Credit: lory_adventures on Instagram.
A bird’s heart is typically twice as heavy as a mammal’s of the same size and is capable of pumping more blood with each beat in order to supply the body with enough oxygen during flight.10 Likewise, a bird’s respiratory system is optimized to meet the oxygen demands of flight. It includes not just lungs, but a series of air sacs. (Source: see footnote 3.) These air sacs increase air intake and allow for the uninterrupted flow of oxygen in and carbon dioxide out. The feathers of flightless dinosaurs probably served display and thermoregulation purposes, but in modern, flighted birds, they also provide key aerodynamic functions, like generating thrust and lift. (Source: see footnote 3.) You may have heard about birds’ exceptional intelligence and ultra-keen vision. Both of these traits are due, at least in part, to the development of flight.11 Their brains had to become more sophisticated in order for them to fly successfully; you can’t move through the air at high speeds without crashing into something unless your eyes and brain can keep up with the incoming visual data. Other adaptations for flight include having a beak (instead of teeth) and a greatly reduced urogenital system, both of which do away with unnecessary weight. (Source: see footnote 3.)
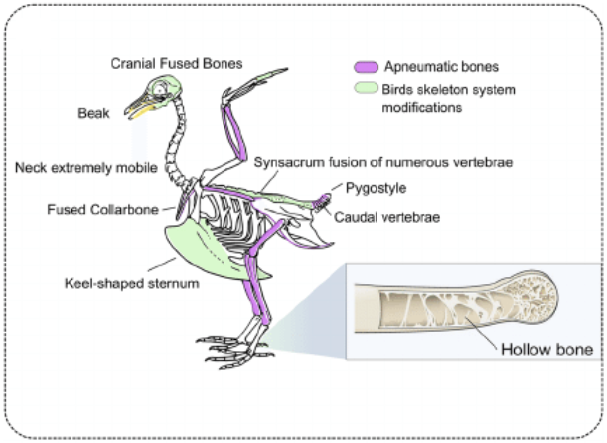
This image, from a research paper on how certain bone-related genes may have contributed to the origin of flight21, highlights some of the skeletal adaptations birds possess which make flight possible.
If you didn’t before, you now understand that a bird’s body is specifically adapted for flight. But there are flightless birds, aren’t there? Yes, there are bird species which have evolved to be flightless. The ostrich and the emu are flightless. The Galápagos cormorant and the kiwi are flightless. Flamingos, for example, are not flightless. They are in fact very strong flyers capable of travelling several hundred miles without stopping, often in a single night.12 These birds migrate to reach breeding grounds and fly from wetland to wetland in order to forage.13 So, if you see them in an outdoor enclosure with no walls or roof and find yourself wondering why they don’t fly away, it’s because they’re either pinioned (a surgical amputation of part of the wing) or their wings are clipped.14 One justification for the pinioning of zoo birds is “giving visitors the impression that the birds are ‘free'” when in reality they have been permanently disabled through amputation.15 Thus, we can be conditioned to see any bird as flightless–even ones that clearly fly a great deal in the wild. If you’ve ever been to a pet store and seen a parrot who sits out in the open and never flies, you may have thought that this is because parrots don’t fly very much or don’t like to fly. But parrots, like flamingos, are natural-born flyers; it is very rare for a captive parrot to be entirely flightless for reasons other than clipped wings or confinement.
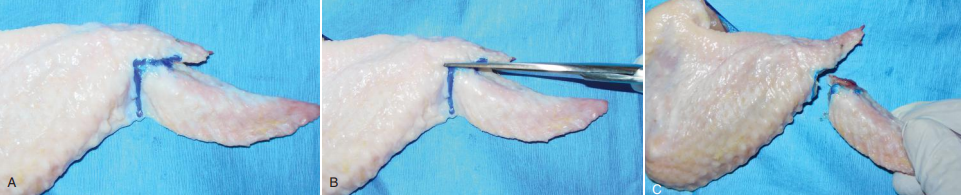
Pinioning, the means by which many captive flamingo populations are rendered flightless, is surgical amputation. Procedures such as pinioning and the clipping of wings change public perception of how birds function in the wild. Images from Current Therapy in Avian Medicine and Surgery.22
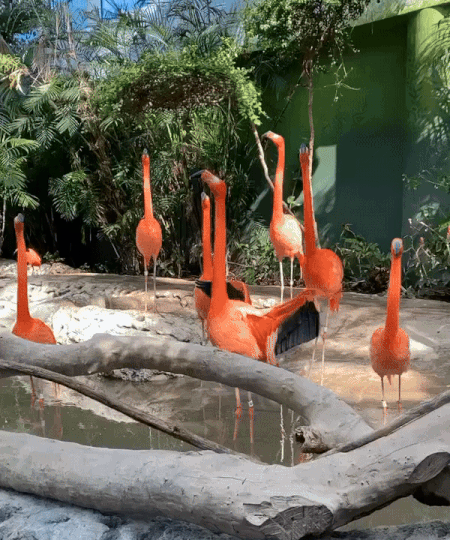
These flamingos (found at a large and popular AZA-accredited zoo in the U.S.) cannot fly because they have each had one of their wings cut in half. The amputation is deliberately performed on only one wing so that they will be imbalanced, making all flight completely impossible.
Did you know that swimming pools are a required component of penguin enclosures even though penguins studied across multiple zoos only used their pools between 2 and 23% of the time?16, 17 Swimming is considered a basic need in penguins and is used as an indicator for physical activity and natural behaviour. (Source: see footnote 17.) Penguins may be flightless in the literal sense, but not in the biomechanical sense. For the purposes of a study on avian locomotion and bone structure, penguins were not grouped with other flightless birds. They were placed in their own group because their wings serve as lift-generating hydrofoils and allow for a type of “aquatic flight”.18 Therefore, a penguin’s version of flight is deemed indispensable, with zoos seeking to encourage it for the betterment of penguin welfare, even while preventing parrots from flying remains standard among many aviculturists and bird owners. “Folklore husbandry”, a term gaining traction in the world of herpetology, refers to methods of keeping animals in captivity that are implicitly accepted and followed out of tradition.19 It would seem that parrots are long-suffering victims of folklore husbandry; they are consistently denied flight, their natural locomotion, because of old wives’ tales, e.g. “birds can’t avoid windows” or “flighted birds are more aggressive”.
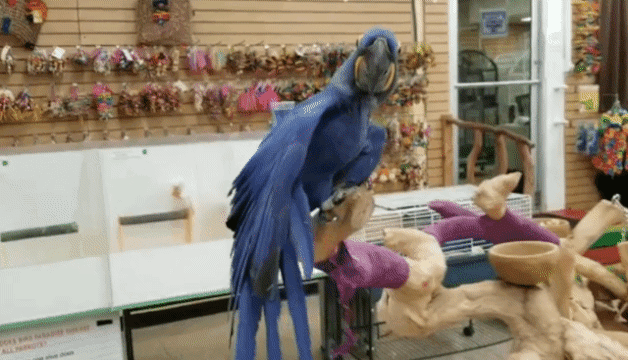
Misconceptions about parrots lead bird owners to accept clipped wings and a sedentary lifestyle as normal. Credit: Anonymous contribution.
Consistent exposure to wing-clipped parrots has managed to convince many people that these are inherently slow-moving, lazy creatures who are content to sit on a branch or a shoulder all day. But parrots, believe it or not, are closely related to and share a common ancestor with falcons. In fact, parrots are more closely related to falcons than falcons are to hawks and eagles.23 (Additional source: see footnote 1.) There is one species of naturally flightless parrot, but you won’t have seen it at any pet shop. It is New Zealand’s critically endangered kakapo, a descendant of another New Zealand parrot, the kea, which itself diverged from all other parrots about 60 million years ago.24 The kakapo evolved to be giant, flightless, and nocturnal because there were no mammalian predators in its environment, but there were some birds of prey who hunted during the day. It has a low metabolism, legs which are longer and stronger than those of flighted parrot species, and a running gait. Its keel is diminished, its breast and wing muscles are reduced, and it has the smallest relative wing size of any parrot.25 It would be illogical to argue, then (as I have seen some do on occasion), that the kakapo’s flightlessness has any bearing on the important role flight plays for our pet birds. The kakapo is unique among parrots, having been shaped by a unique environment; to argue that a blue and gold macaw need not fly because the kakapo does not fly makes about as much sense as arguing that a neotropic cormorant need not fly because the flightless cormorant does not fly.
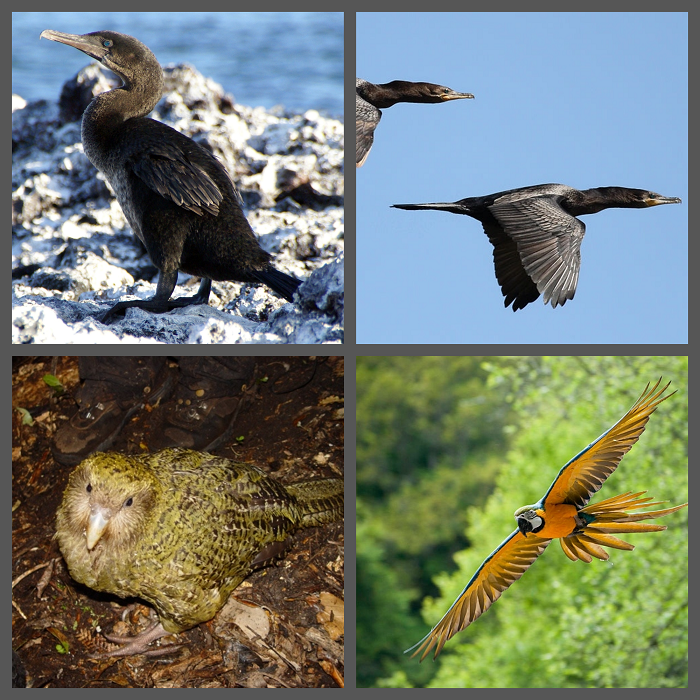
Different bird species are adapted to different lifestyles. We wouldn’t clip cormorants if we kept them as pets…would we? Images from Wikimedia Commons.35
Just as the kakapo is born to running and climbing, the parrot species we keep as pets are born to flight. Macaws, for example, are recognised as having a major influence on the Amazonian ecosystem because they are strong flyers who disperse fruit seeds over long distances.26 It would be impractical, of course, to discuss each and every type of parrot here. Instead, I present you with two quotations from two different scientific journal publications which comment on parrots in general: 1.) “…most parrots fly over large areas within a given day”27 and 2.) “We measured the distances that fruits or seeds were carried by parrots… Unsurprisingly, given the long-distance flying abilities of most parrot species, the distances spanned a wide range.”28 Budgerigars and cockatiels are common test subjects in studies of avian flight mechanics and metabolism. For the purposes of many experiments, they are trained to fly in wind tunnels so that scientists can gather reliable data under controlled conditions.29, 30, 31, 32 These birds would not be chosen again and again for wind tunnel experiments examining flight if they were weak flyers.
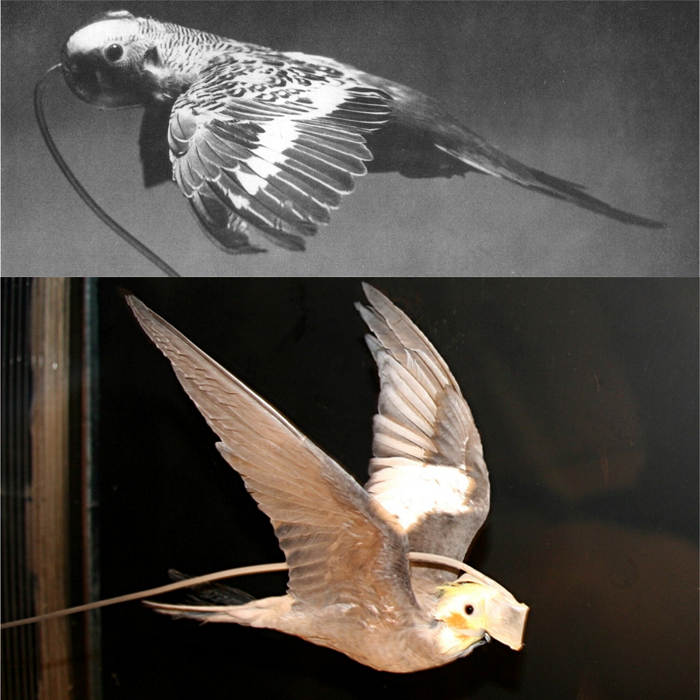
Studies on parrots yield valuable information about avian flight biology. Budgie image by Vance Tucker (see footnote 29) and cockatiel image by Graham Askew.36
Finally, parrots are prey, and they have their flight skills put to the test by the animals that hunt them.33 If parrots didn’t want to fly, they wouldn’t be able to survive. Your parrot may be safe from predators, but the instinct and urge to fly, written into its genes over the course of millions of years, remains. A study on the welfare of birds in captivity concluded that birds are “highly inclined to fly as part of essential locomotion even though basic needs are provided”.34 This study, though lacking in statistical analysis, is one of very few which have endeavoured to explore the effects of flight deprivation/restriction on naturally flighted birds. Because flight deprivation/restriction is seen as permissible or even standard practice in the management of many captive bird species, this topic might be something of a bogeyman many researchers would rather not touch. On the other hand, when an animal has evolved to use flight as its predominant means of locomotion, is a study truly needed to prove that flight should be an indispensable facet of its life in captivity? If swimming is considered a basic need for penguins, how can it be that flight is not considered a basic need for parrots? How can it be that clipping wings is still standard at so many establishments? We do not perform studies on penguins where they are prevented from swimming their entire lives just to prove that swimming is of great biological importance to them. There simply is no argument, aside from one made in the name of human convenience at the expense of animal welfare, which can withstand this disparity.
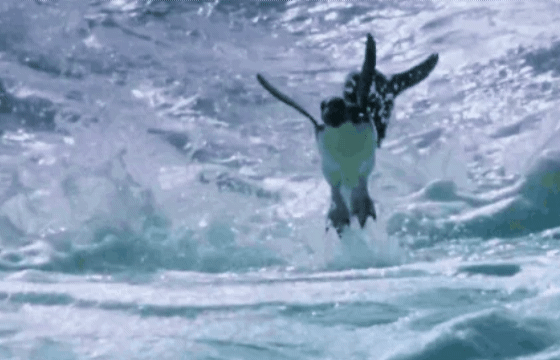
Swimming, in the case of penguins, can be considered a form of “aquatic flight”. Penguins in captivity are not deprived the ability to swim. Even though they cannot swim the open seas, they are still given pools to exercise in.
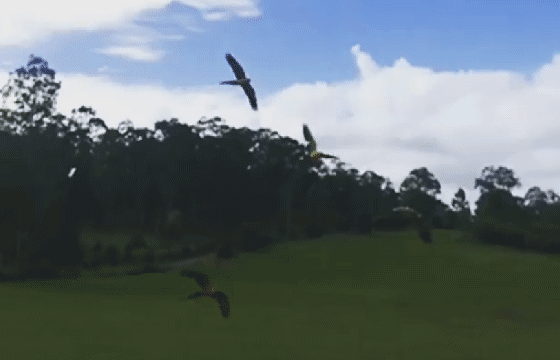
These captive parrots, who have been specially prepared and trained for outdoor flight, are not fleeing from predators or travelling in search of food–nor are they forced by a human to fly. They fly because it is an inherently rewarding and entirely natural behaviour. Credit: @flightedfeathers on Instagram.
Even after accepting that parrots are built for flight, we are still left with unanswered questions concerning people’s pets. Why do some bird owners say that their parrots have no interest in flying and never try to fly? The answer can typically be found somewhere in the bird’s history. It may have been wing-clipped or confined to a cage during early development and never learned how to fly, it may have had its wings clipped for such a long time that its muscles have atrophied, it may experience psychological reluctance to try and fly due to previously falling and crashing with clipped wings and/or atrophied muscles, and so on. These same birds who appear flightless under ordinary circumstances still instinctively respond to sudden frights by attempting to fly, which is why you can lose a bird who has been clipped for years by bringing it outdoors unrestrained.
Conversely, why do some people say that their parrots can still fly “just fine” while clipped? This may be because their birds happen to have very conservative wing-clips, but it can also be attributed to the size of the bird. Owners of clipped medium and large parrots tend to say that their birds don’t mind being flightless because they never try to fly, while owners of clipped small parrots tend to say that their birds fly “pretty well”. The truth is that it is typically much more difficult for a large bird with clipped wings to fly, while a small bird with the same clip may still be capable of some short-distance flight. This has nothing to do with differences in how much small versus large parrots fly naturally but everything to do with the aerodynamic concept of wing loading.
Wing loading is a measurement of how much weight a wing is carrying per unit area. A budgie’s is about 2.7 kg/m^2, a cockatiel’s is about 2.4 kg/m^2, and a blue and gold macaw’s is about 8.3 kg/m^2.37, 38 Thus, a blue and gold macaw’s wing carries just over three times as much weight per square metre as a budgie’s. Reduce the wing’s surface area by cutting feathers and it becomes even higher. This is why small birds are more likely to learn to partially compensate for their disability while large birds are more likely to remain grounded or simply find flight attempts–and the resulting crashes–too punishing.
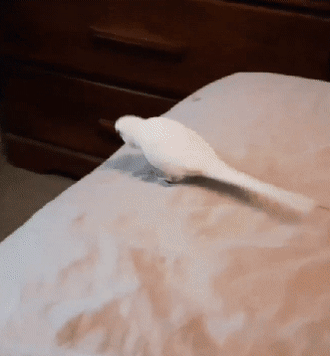
This severely clipped budgie launches itself in an upward arc and makes it from the bed to the window. This bird is a poor flyer with no appreciable ability to turn or land properly, but it can accomplish more forward flight than the galah pictured at right. Credit: Jennifer Koch.
As you can see, clipping wings has complex and far-reaching implications. Clipping may lead to flight attempts that result in injury and stress, flight attempts that result in partially compensating for a disability, or no flight attempts at all and a lapse into a totally flightless lifestyle. If the clip is performed before or during a sensitive period of development, it can cause detriments that prove incredibly difficult to overcome.
Why is it so important for a bird to learn how to fly as a baby? Click here to find out!
References
- Brusatte, S.L., O’Connor, J.K. and Jarvis, E.D., 2015. The origin and diversification of birds. Current Biology, 25(19), pp. R888-R898. https://www.sciencedirect.com/science/article/pii/S0960982215009458
- Clarke, J.A., Tambussi, C.P., Noriega, J.I., Erickson, G.M. and Ketcham, R.A., 2005. Definitive fossil evidence for the extant avian radiation in the Cretaceous. Nature, 433(7023), p.305. https://www.nature.com/articles/nature03150
- Morrison, M.L., Rodewald, A.D., Voelker, G., Colón, M.R., Prather, J.F., 2018. Ornithology: Foundation, Analysis, and Application. JHU Press, p. 6. https://books.google.com/books/about/Ornithology.html?id=jN5wDwAAQBAJ&source=kp_book_description
- Dumont, E.R., 2010. Bone density and the lightweight skeletons of birds. Proceedings of the Royal Society B: Biological Sciences, 277(1691), pp.2193-2198. https://royalsocietypublishing.org/doi/full/10.1098/rspb.2010.0117
- Sullivan, T.N., Meyers, M.A. and Arzt, E., 2019. Scaling of bird wings and feathers for efficient flight. Science advances, 5(1), p.eaat4269. https://advances.sciencemag.org/content/5/1/eaat4269
- Biewener, A.A., 2011. Muscle function in avian flight: achieving power and control. Philosophical Transactions of the Royal Society B: Biological Sciences, 366(1570), pp.1496-1506. https://royalsocietypublishing.org/doi/full/10.1098/rstb.2010.0353
- Zheng, X., Wang, X., O'connor, J. and Zhou, Z., 2012. Insight into the early evolution of the avian sternum from juvenile enantiornithines. Nature Communications, 3, p.1116. https://www.nature.com/articles/ncomms2104?WT=
- Janssen, I., Heymsfield, S.B., Wang, Z. and Ross, R., 2000. Skeletal muscle mass and distribution in 468 men and women aged 18–88 yr. Journal of applied physiology, 89(1), pp.81-88. https://www.physiology.org/doi/full/10.1152/jappl.2000.89.1.81
- Patak, A. and Baldwin, J., 1993. Structural and metabolic characterization of the muscles used to power running in the emu (Dromaius novaehollandiae), a giant flightless bird. Journal of Experimental Biology, 175(1), pp.233-249. https://jeb.biologists.org/content/175/1/233.short
- Pees, M. and Krautwald-Junghanns, M.E., 2009. Cardiovascular physiology and diseases of pet birds. Veterinary Clinics of North America: Exotic Animal Practice, 12(1), pp.81-97. https://www.sciencedirect.com/science/article/abs/pii/S1094919408000583
- Shimizu, T., Shinozuka, K., Uysal, A.K. and Kellogg, S.L., 2017. The origins of the bird brain: multiple pulses of cerebral expansion in evolution. In Evolution of the Brain, Cognition, and Emotion in Vertebrates (pp. 35-57). Springer, Tokyo. https://link.springer.com/chapter/10.1007/978-4-431-56559-8_2
- Amat, J.A., Rendón, M.A., Rendón-Martos, M., Garrido, A. and Ramírez, J.M., 2005. Ranging behaviour of greater flamingos during the breeding and post-breeding periods: linking connectivity to biological processes. Biological Conservation, 125(2), pp.183-192. https://www.sciencedirect.com/science/article/abs/pii/S0006320705001436
- McCulloch, G., Aebischer, A. and Irvine, K., 2003. Satellite tracking of flamingos in southern Africa: the importance of small wetlands for management and conservation. Oryx, 37(4), pp.480-483. https://www.cambridge.org/core/journals/oryx/article/satellite-tracking-of-flamingos-in-southern-africa-the-importance-of-small-wetlands-for-management-and-conservation/431FE98B97C58B3B2CC4757E8146B734
- Norton, T.M. et al., n.d. Flamingo Health and Medicine Program. American Association of Zoo Veterinarians. Available at: https://www.aazv.org/page/flamingo_health_med_ (Accessed: 29 June 2019).
- NSW Guidelines for the pinioning of birds, n.d. Department of Primary Industries, New South Wales Government, Australia. https://www.dpi.nsw.gov.au/animals-and-livestock/animal-welfare/general/bird-pinioning
- AZA Penguin Taxon Advisory Group, 2014. Penguin (Spheniscidae) Care Manual. Silver Spring, MD: Association of Zoos and Aquariums. Available at: https://www.speakcdn.com/assets/2332/penguin_care_manual_aza_final_2014.pdf (Accessed: 29 June 2019).
- Marshall, A.R., Deere, N.J., Little, H.A., Snipp, R., Goulder, J. and Mayer‐Clarke, S., 2016. Husbandry and enclosure influences on penguin behavior and conservation breeding. Zoo biology, 35(5), pp.385-397. https://www.researchgate.net/publication/305687885_Husbandry_and_enclosure_influences_on_penguin_behavior_and_conservation_breeding
- Habib, M.B. and Ruff, C.B., 2008. The effects of locomotion on the structural characteristics of avian limb bones. Zoological Journal of the Linnean Society, 153(3), pp.601-624. https://academic.oup.com/zoolinnean/article/153/3/601/2606425
- Arbuckle, K., 2013. Folklore husbandry and a philosophical model for the design of captive management regimes. Herpetol Rev, 44(5), pp.448-452. https://www.researchgate.net/profile/Kevin_Arbuckle/publication/262116292_Folklore_husbandry_and_a_philosophical_model_for_the_design_of_captive_management_regimes/links/00463536b55c1e7249000000.pdf
- Jaggard, V., Clark, R., 2018. These Are the Dinosaurs That Didn't Die. National Geographic Magazine. https://www.nationalgeographic.com/magazine/2018/05/dinosaurs-survivors-birds-fossils/
- Machado, J.P., Johnson, W.E., Gilbert, M.T.P., Zhang, G., Jarvis, E.D., O’Brien, S.J. and Antunes, A., 2016. Bone-associated gene evolution and the origin of flight in birds. BMC genomics, 17(1), p.371. https://link.springer.com/article/10.1186/s12864-016-2681-7
- Speer, B., 2015. Current therapy in avian medicine and surgery. Elsevier Health Sciences, p. 694. https://books.google.com/books?id=LKY_CwAAQBAJ&source=gbs_navlinks_s
- Prum, R.O., Berv, J.S., Dornburg, A., Field, D.J., Townsend, J.P., Lemmon, E.M. and Lemmon, A.R., 2015. A comprehensive phylogeny of birds (Aves) using targeted next-generation DNA sequencing. Nature, 526(7574), p.569. https://www.nature.com/articles/nature15697
- Schweizer, M., Seehausen, O. and Hertwig, S.T., 2011. Macroevolutionary patterns in the diversification of parrots: effects of climate change, geological events and key innovations. Journal of Biogeography, 38(11), pp.2176-2194. https://onlinelibrary.wiley.com/doi/abs/10.1111/j.1365-2699.2011.02555.x
- Powlesland, R.P., Merton, D.V. and Cockrem, J.F., 2006. A parrot apart: the natural history of the kakapo (Strigops habroptilus), and the context of its conservation management. Notornis, 53(1), p.3. https://www.notornis.osnz.org.nz/system/files/Notornis_53_1_3.pdf
- Baños-Villalba, A., Blanco, G., Díaz-Luque, J.A., Dénes, F.V., Hiraldo, F. and Tella, J.L., 2017. Seed dispersal by macaws shapes the landscape of an Amazonian ecosystem. Scientific reports, 7(1), p.7373. https://www.nature.com/articles/s41598-017-07697-5
- Gilardi, J.D. and Munn, C.A., 1998. Patterns of activity, flocking, and habitat use in parrots of the Peruvian Amazon. The Condor, 100(4), pp.641-653. https://academic.oup.com/condor/article/100/4/641/5126022
- Tella, J.L., Baños-Villalba, A., Hernández-Brito, D., Rojas, A., Pacífico, E., Díaz-Luque, J.A., Carrete, M., Blanco, G. and Hiraldo, F., 2015. Parrots as overlooked seed dispersers. Frontiers in Ecology and the Environment, 13(6), pp.338-339. https://esajournals.onlinelibrary.wiley.com/doi/full/10.1890/1540-9295-13.6.338
- Tobalske, B.W., 2007. Biomechanics of bird flight. Journal of Experimental Biology, 210(18), pp.3135-3146. https://jeb.biologists.org/content/210/18/3135
- Bundle, M.W., 2009. Oxygen uptake of flying budgerigars by VA Tucker. Journal of Experimental Biology, 212(22), pp.3595-3596. https://jeb.biologists.org/content/212/22/3595
- Hedrick, T.L., Tobalske, B.W. and Biewener, A.A., 2003. How cockatiels (Nymphicus hollandicus) modulate pectoralis power output across flight speeds. Journal of Experimental Biology, 206(8), pp.1363-1378. https://jeb.biologists.org/content/206/8/1363
- Morris, C.R., Nelson, F.E. and Askew, G.N., 2010. The metabolic power requirements of flight and estimations of flight muscle efficiency in the cockatiel (Nymphicus hollandicus). Journal of Experimental Biology, 213(16), pp.2788-2796. https://jeb.biologists.org/content/213/16/2788.full
- Brightsmith, D.J., 2002. What Eats Parrots? Bird Talk Magazine. http://vetmed.tamu.edu/macawproject/wp-content/uploads/sites/45/2019/03/what_eats_parrots_2002_aug.pdf
- Peng, S.J.L., Chang, F.C., Sheng-Ting, J.I. and Fei, A.C.Y., 2013. Welfare assessment of flight-restrained captive birds: effects of inhibition of locomotion. The Thai Journal of Veterinary Medicine, 43(2), p.235. https://www.researchgate.net/publication/287605561_Welfare_Assessment_of_Flight-restrained_Captive_Birds_Effects_of_Inhibition_of_Locomotion
- Flightless cormorant: https://commons.wikimedia.org/wiki/File:Flightless_cormorant_(Phalacrocorax_harrisi)_-Isabela.jpg; Neotropic cormorant: https://commons.wikimedia.org/wiki/File:Phalacrocorax_brasilianus_-Argentina_-two_flying-8.jpg; Kakapo: https://commons.wikimedia.org/wiki/File:Strigops_habroptilus_1.jpg; Blue and gold macaw: https://commons.wikimedia.org/wiki/File:Ara_ararauna_-Zoo_de_Pont-Scorff,_Morbihan,_France-8a_(2).jpg
- Knight, K., 2010. How birds power flight. Journal of Experimental Biology, 213(16), pp. i-ii. https://jeb.biologists.org/content/213/16/i.short
- Tobalske, B.W., Hedrick, T.L. and Biewener, A.A., 2003. Wing kinematics of avian flight across speeds. Journal of Avian Biology, 34(2), pp.177-184. https://citeseerx.ist.psu.edu/viewdoc/download?doi=10.1.1.511.9726&rep=rep1&type=pdf
- Bhargavi, S., Venkatesan, S., Ramesh, G. and Kannan, T.A., 2017. Morphometrical Analysis of the Wing of the Blue-And-Yellow Macaw (Ara ararauna) With Reference to the Aerodynamics. International Journal of Current Microbiology and Applied Sciences, 6(8), pp. 2707-2710. https://www.ijcmas.com/6-8-2017/S.%20Bhargavi,%20et%20al.pdf